Pests pose a significant challenge to the continued productivity, profitability, and sustainability of aquaculture. There has been a lot of research undertaken in NZ and overseas to develop pest control techniques for aquaculture, but this has rarely translated into useable options for farmers. We believe that the lack of implementation is because we lack the context of how, when, and where to apply pest control techniques for positive on-farm outcomes. This conundrum has been tackled in terrestrial agriculture, for example via the concept of Integrated Pest Management (IPM), which uses ecology and risk assessment to define optimal pest management strategies that employ available control techniques. This concept can absolutely be translated to aquaculture, and has many potential benefits (see review of Aquaculture-IPM here).
There are five key pillars of Aquaculture-IPM:
- Pest ecology – understanding which conditions favour pest outbreaks allows for targeted control strategies.
- Bioeconomic cost-benefit – there are different biological and financial costs (and benefits) of different management strategies.
- Continual monitoring – early detection and intervention is an important factor in pest management.
- Proactive prevention – steps can be taken to prevent pest problems before they start, rather than reacting to them after they occur.
- Reactive control – there are a range of chemical and physical methods to manage pests when they do occur.
ShARP researchers are working on several projects to develop an effective IPM strategy for common pests in bivalve aquaculture. We are currently developing IPM for two priority pests in NZ shellfish aquaculture and are also developing innovative tools to support its implementation more broadly.
1. Cladophora blooms on mussel farms in the Marlborough Sounds
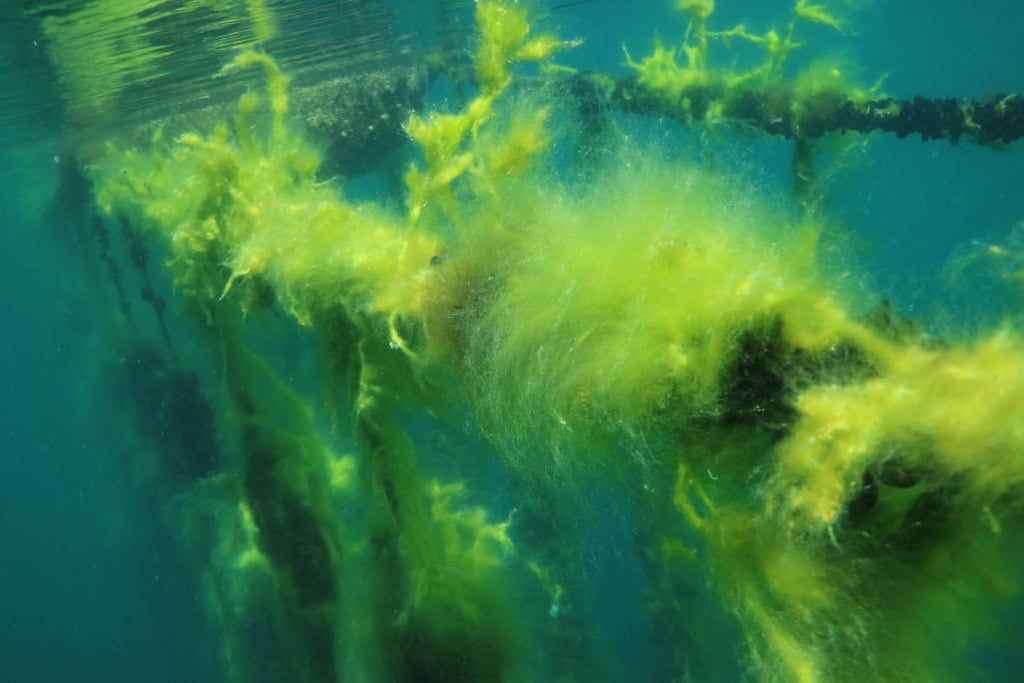
Cladophora ruchingeri is a seaweed (macroalga) which grows on mussel ropes in the Marlborough Sounds
Cladophora is genus of green algae which contains many different species. Blooms of the species Cladophora ruchingeri (also known as ‘horse hair’) grow on mussel farms in the Marlborough Sounds. When its settlement and growth reach a high intensity, Cladophora both competes with mussels for space and nutrients, and clogs harvesting equipment – resulting in costly delays, and stock losses.
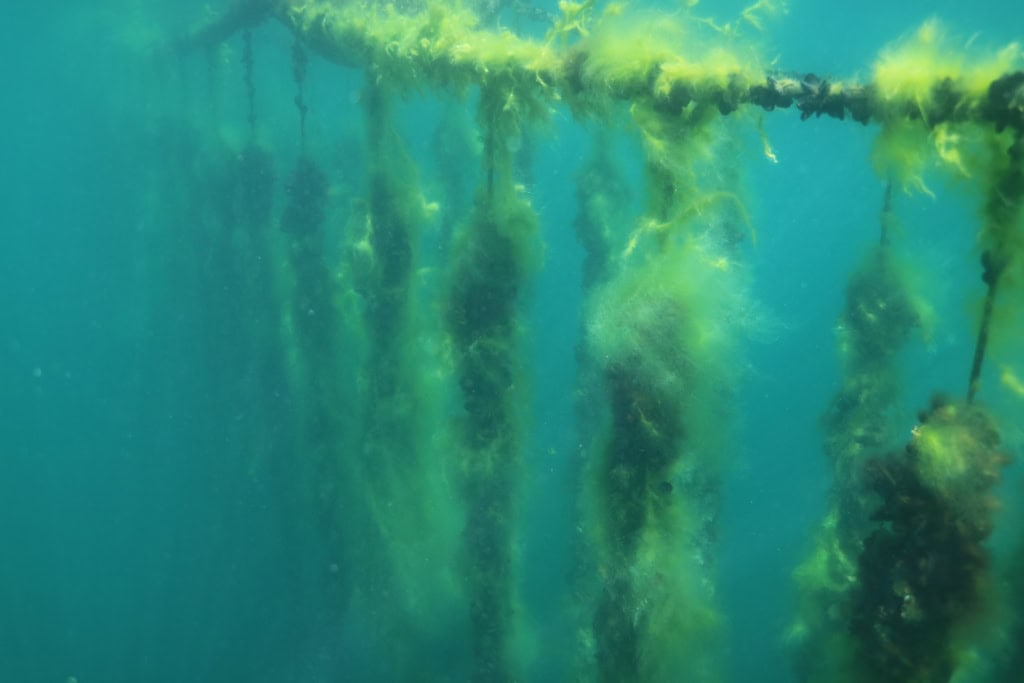
Above, Cladophora on mussel lines
ShARP researchers, led by Cawthron Scientist Dr Malindi Gammon are working to understand:
- How Cladophora settles and grows on mussel lines
- The direct and indirect effects of Cladophora on farmed mussels (i.e., direct effects may be e.g. competing for space, and indirect effects may be e.g. reducing the light and nutrients available to mussels)
- What causes Cladophora to ‘bloom’
- What treatments have the potential to reduce or remove Cladophora, without negatively impacting mussels
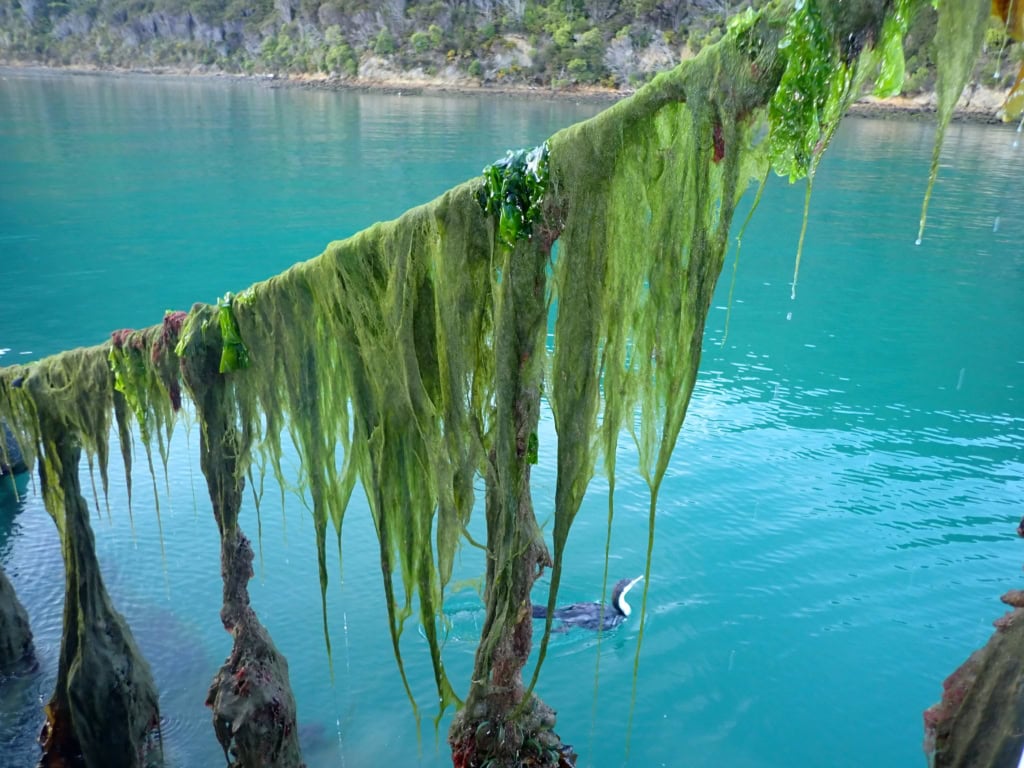
Above, in its mature state Cladophora ruchingeri forms characteristically long strands or fronds which look like ‘horse-hair’ when the mussel lines are lifted. Photo: M Gammon
Collectively this information will inform strategies to avoid or manage the operational impacts of Cladophora.
Potential strategies could include:
- Monitoring mussel lines for early signs of Cladophora and applying a treatment (e.g. acetic acid/vinegar) to destroy any early colonizers.
- Lowering the depth of mussel lines to reduce the amount of light reaching them so that Cladophora cannot flourish. This is a method known as ‘subbing’.
By identifying high-risk areas and periods for Cladophora blooms, potential strategies can be targeted to the locations and times when they will be most effective.
2. Bivalve-eating flatworms
Predatory flatworms consume juvenile and adult shellfish, and are an issue for aquaculture around the world. Cawthron researchers have shown that at acetic acid ‘dip’ (similar to household vinegar) can reliably kill flatworms while not affecting the shellfish. Read our recent paper on this topic here. However, infestations are often only identified when adult flatworms are observed in dead and empty shells, and a substantial amount of stock loss may have already occurred.
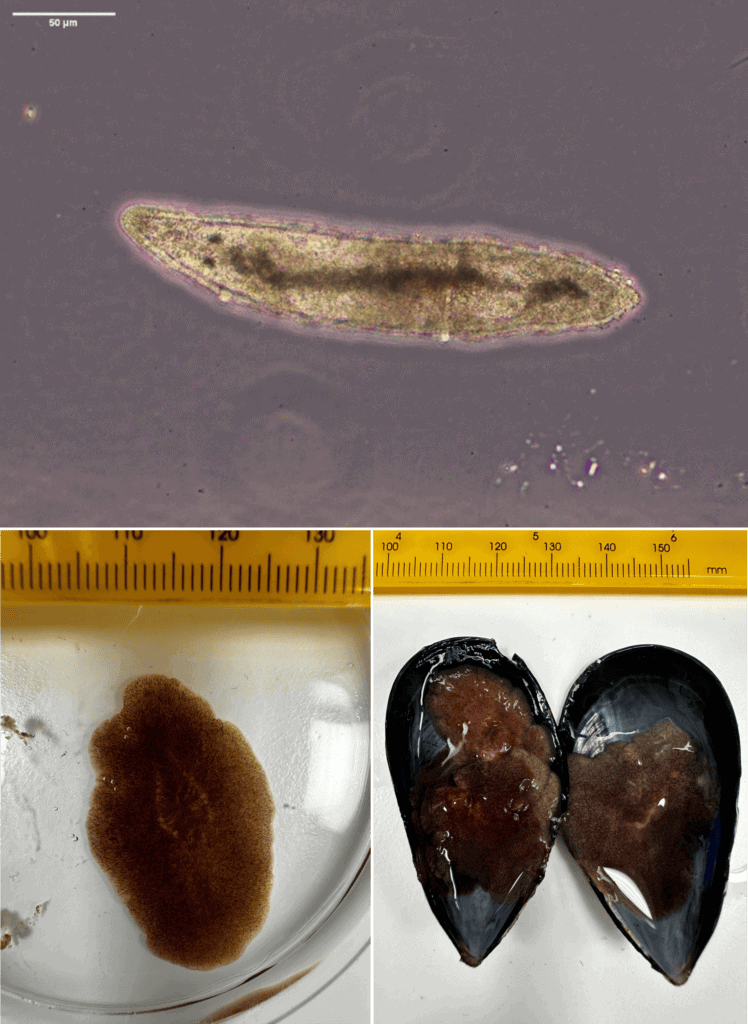
Although adult flatworms can be seen with the naked-eye, early larval life stages are microscopic, making early detection of infestations very difficult. The top image shows a larval flatworm (Postenterogonia orbicularis); bottom left shows an adult of the same species on a petri dish beside a mm scale ruler, and bottom right, a blue mussel showing the final fatal effects of an infestation of this species. Photos: M Gammon, Cawthron.
There are a number of species responsible for bivalve predation in New Zealand. One common species is the oyster-eating flatworm Postenterogonia orbicularis and Cawthron researchers led by Dr Malindi Gammon are working to understand:
- different stages in its lifecycle,
- how environmental parameters, like temperature, impact the speed at which they go through their life cycle, and how this might lead to a population ‘boom’
- how populations settle and establish on farms and whether certain operational practices might ‘encourage’ or ‘discourage’ flatworm larvae to settle, includes understanding the role of light.
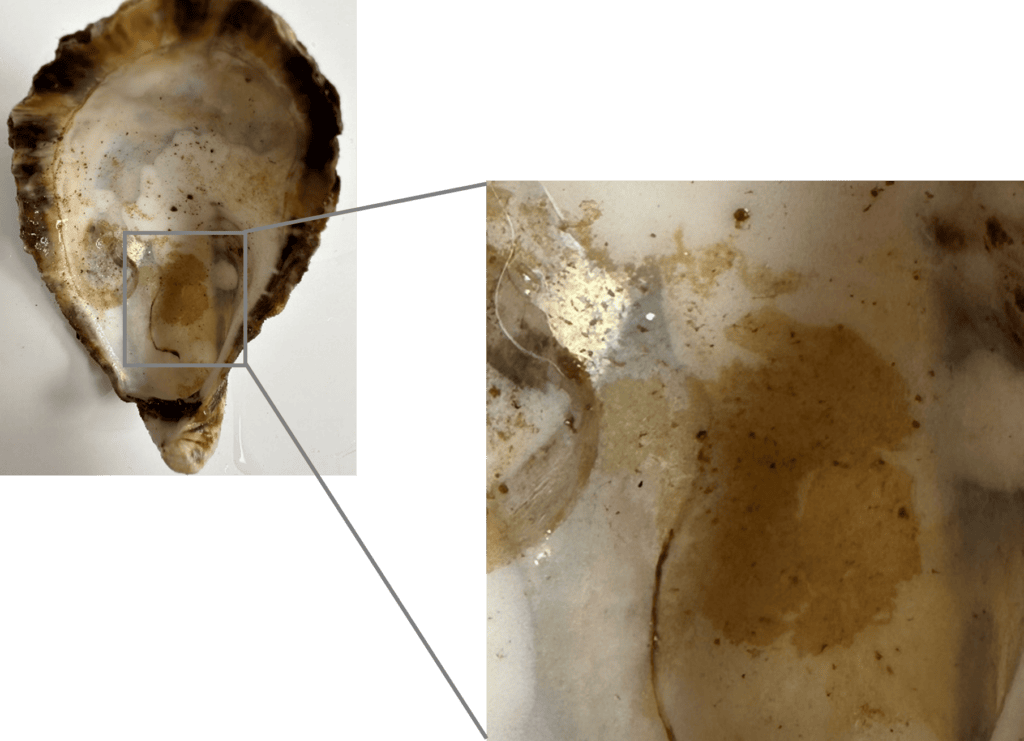
Above: Flatworms can lay hundreds to thousands of eggs at a time. While the individual eggs are hard to detect, they lay eggs in a continuous ‘egg-plate’ which appears as a yellowish mark – typically on the inside of dead and empty shellfish shells. In this case the shell of a Pacific oyster. Photo: M Gammon, Cawthron.
This information will help identify ‘high-risk’ periods for flatworm infestations on oyster farms, and inform potential intervention strategies.
Potential strategies could include:
- Monitoring oyster stock during high-risk periods to detect and destroy flatworm eggs before they hatch.
- Minimising the types of surfaces that flatworms use for laying eggs, including smooth, hard surfaces, such as the insides of empty oyster shells. Timely removal of dead oysters from baskets during high-risk periods might help reduce the likelihood of an infestation.
- Depending on the role of light in larvae settlement, light could be manipulated to ensure larvae aren’t ‘attracted’ to a farm (for example, by shading baskets during the day or lighting them at night).
3. Camera technologies for real-time detection of biofouling settlement events
The settlement and growth of non-target marine organisms (e.g., barnacles, sea squirts) on farm structures is a persistent issue for the green-lipped mussel (Perna canaliculus) and wider shellfish aquaculture industry. Accumulation of this growth – termed biofouling – on culture equipment (e.g., mussel ropes) reduces the space and nutrients available to farmed stock, limiting production, and creates additional costs for operators associated with its removal.
Being able to anticipate and readily detect when settlement events of larvae of these non-target organisms occur is important for shellfish farmers to be able to undertake rapid and effective action to prevent their establishment on farm infrastructure. Early interventions (e.g., temporarily sinking mussel lines to avoid larval pulse) will reduce the impacts of these organisms on farm productivity as well as the labour and costs associated with their management, as current measures are carried out when growth is already well-established.
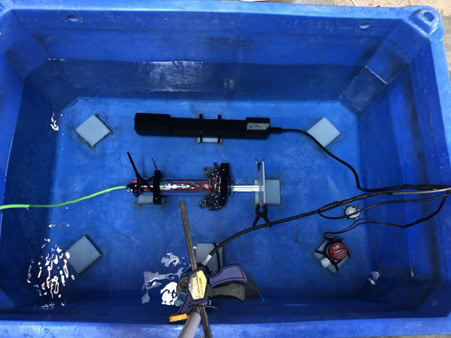
Experiment to optimise detection limits for a camera prototype and setup for deployment under field conditions on farms.
To enable this, a team of Cawthron researchers led by Dr Bailey Lovett are developing an on-farm ‘sentinel’ camera system to detect ‘pulse’ settlement events of larvae of common nuisance biofouling taxa (e.g., blue mussels, sea squirts, barnacles) in real-time. While similar technologies are currently available, such models typically cost in the order of hundreds of thousands of dollars, which is not economically feasible for many shellfish operators. The aim of the current project is therefore to develop an affordable solution that is practical, user-friendly, and fit-for-purpose. The technology will also provide a valuable experimental tool for biosecurity scientists and ecologists more broadly to monitor patterns in species biodiversity, distribution, and propagule pressure in the marine environment. At this stage of the project, researchers are optimising detection limits for the current camera prototype and setup (image on left) for deployment under field conditions (i.e., on the farms). Trials to understand detection sensitivity under environmentally-relevant conditions of turbidity (e.g. from silt or algal blooms) have recently been completed. A further series of lab-based trials is currently underway to understand biologically-relevant timeframes, and to optimise camera system parameters for detection of the larvae of relevant biofouling species such as sea squirts and blue mussels.
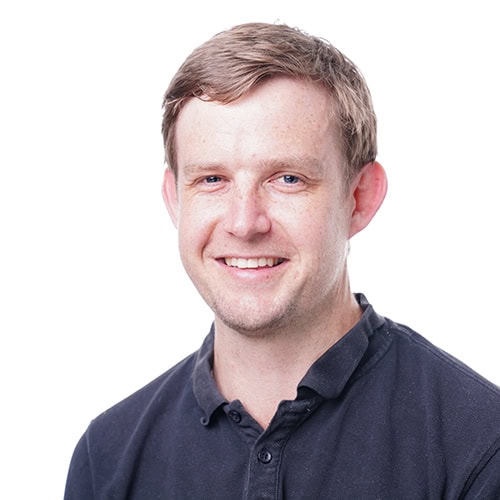
Contact Dr Patrick Cahill
Manager – Biosecurity, Cawthron Institute